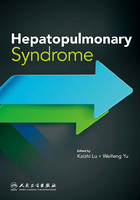
上QQ阅读APP看书,第一时间看更新
Pathogenesis of HPS
Cirrhosis and portal hypertension result in the deleterious effects on multiple organs, thus increasing morbidity and mortality 3. In particular, the diseased liver-induced lung dysfunctions occur as a result of series changes, such as the decreased hepatic clearance ability, or the increased production of circulating cytokines and other mediators. These circulating factors target pulmonary vasculature and cause IPVD and intrapulmonary shunts, thereby leading to arterial oxygenation defect and hypoxemia. Hypoxic conditions contribute to the proliferation of pulmonary arterial smooth muscle cells (PASMCs) involved in vascular remodeling, thus aggravating the progression of HPS, as shown in Figure 1. Furthermore, the pathophysiology of HPS may involve more other factors in addition to IPVD. For example, the increased pulmonary capillary density and vascular remodeling in the pulmonary vasculature have been recognized as a key component in the development of HPS 4,5. Because the pathogenesis of HPS is not fully understood, the development of effective medical therapies is very limited. Thus, it is necessary to establish a strategy to treat HPS based on the study of HPS pathogenesis.

Figure 1. The development and progression of HPS
Here, we will describe several important pathogeneses of HPS.
IPVD and intrapulmonary shunting
IPVD, the most well described alteration in HPS, is characterized by the dilation of precapillary and postcapillary in the pulmonary vasculature. Increased production of vasodilators,decreased clearance of vasodilators, or decreased production of vasoconstrictors, all emanating from diseased liver, might impair the balance between vasoconstriction and vasodilation in pulmonary vasculature. Early studies revealed obvious dilation of capillary through the method of injection at autopsy with cirrhosis. Later studies have confirmed an increase of pulmonary diameter as the major structural alteration in HPS. The diameter of pulmonary capillaries normally reaches 15 μm in healthy individuals, whereas in HPS patients pulmonary capillaries are signifcantly enlarged to 15-60 μm. In some cases, pulmonary capillaries can increase up to 500 μm, predominately in the lung bases. Capillary dilation makes a larger distance between erythrocytes and alveolar wall. Venous blood, when passing through the dilated capillary in the lung, have a much faster flow speed, which leads to the more difficult oxygenation of rapidly passing erythrocytes. In addition, increased pulmonary arteriovenous communications and venous anastomoses have been found in the pulmonary vasculature 6. And thickened pulmonary capillaries have also been observed 7. Arterial hypoxemia induced by intrapulmonary shunt occurs as a result of the mixture of oxygenated venous blood with deoxygenated venous blood.
IPVD enhances the passage of mixed venous blood either rapidly or even directly, through intrapulmonary shunting into the pulmonary veins. Ventilation-perfusion mismatch, which is identifed by increased pulmonary blood fow accompanied with decreased alveolar ventilation,lead to the oxygenation defect (Figure 2). Moreover, increased pulmonary blood flow can block hypoxic pulmonary vasoconstriction (HPV) but not cause pulmonary vasoconstriction, in association with alveolar hypoxia, which has been found in 35% of patients with cirrhosis 12.

Figure 2. Mechanism of HPS arterial hypoxemia: a two-compartment model of gas exchange in lung
Hypoxemia
Hypoxemia, a key risk factor for the HPS prognosis, is secondary to IPVD and intrapulmonary shunting. In general, the severity of hypoxemia appears to be directly associated with intrapulmonary shunting, ventilation-perfusion mismatch, or both of them,while portopulmonary vascular communications play a minor role in the process 13-15. In brief,ventilation-perfusion mismatch and intrapulmonary shunting are the pathophysiological mechanisms of orthodeoxia in HPS patients. A stabilized pulmonary vascular tone, which is unlikely to adjust the gravitational perfusion changes to ventilation, can explain the issue 5. When the HPS patients breathe 100% oxygen, the partial pressure of oxygen is increased 16. This case may be related with the increased cardiac output, indicating that mechanism underlying the gasexchange abnormalities in HPS is complicated. The limited ability of alveolar-capillary oxygen diffusion, an indicator of the diffusion-perfusion dysfunction 17, mainly occurs in the advanced stages of HPS. In these stages, high cardiac output shortens the transit time of erythrocytes in the pulmonary circulation, which is similar to a low diffusing capacity mediated by liver dysfunction 18, 13. Because of the incomplete equilibration of carbon monoxide with hemoglobin resulting from the narrow alveolar-capillary interface, the diffusing capacity may be reduced.
In 1970s, low arterial oxygen saturation has been found in patients with cirrhosis. As known, low arterial oxygen saturation is attributed to one or more of the following mechanisms:depression in the affinity of oxyhaemoglobin, intrapulmonary and portopulmonary shunting,limitation of alveolar-capillary oxygen diffusion, and ventilation-perfusion (VA/Q) mismatch,increased closing volume or absence of HPV 19, 20. Meanwhile, a rightward shift in the oxygen dissociation curve often occurs in patients with cirrhosis. Increased concentration of 2,3-diphosphoglycerate within red blood cells results in the depression of affinity between haemoglobin and oxygen. However, this right shift of the oxyhaemoglobin dissociation curve alone cannot explain the status of arterial hypoxaemia. Cigarette smoking, a common habit of patients with alcoholic cirrhosis, can induce closing volume increase, peripheral airways dysfunction and airway obstruction, therefore diminishing carbon monoxide diffusing capacity(TLCO) and causing VA/Q mismatch. Until now, there is still no method to distinguish intrapulmonary shunting, VA/Q mismatch, and diffusion limitation. The available approaches are based on the application of the respiratory gases, TLCO, or radioactive tracers, however none of them are capable of distinguishing these intrapulmonary determinants of hypoxaemia. In addition, using high inspired oxygen fractions to assess intrapulmonary shunting may alter both the bronchial and the pulmonary vascular tone, which may affect pulmonary gas exchange and thus lead to a different result from that obtained with the breathing air of patients.
As the progress of methodology, inert gas elimination technique has been applied in the detection of pulmonary gas exchange 21. This technique is capable of estimating the functional distribution of VA/Q ratios in the lung, based on the elimination pattern of dissolved infused inert gases with different solubility. It also can conduct a computation of PaO 2 based on the amount of VA/Q mismatch and intrapulmonary shunting if no limitation of diffusion, portopulmonary shunts or substantial pulmonary parenchymal oxygen uptake are present. Thus, alveolar-capillary disequilibrium of oxygen makes the predicted PaO 2 level higher than that of the measured PaO 2.
Table 2. The value of arterial oxygen tension in HPS patients from various studies

Table 2: Principal determinants of arterial oxygen tension (PaO 2) in patients with HPS using the multiple inert gas elimination technique (values represent means), from Rodriguez-Roisin, R,Thorax, 0040-6376
This technology has been used by five independent studies to assess the effect of VA/Q relationship on pulmonary gas exchange in patients with liver cirrhosis and various degrees of abnormal gas exchange, the PaO 2 levels ranging from normal to extremely low 12, 14, 22, as shown in table 2. In the first study, Rodrigues-Roisin and their colleagues found the VA/Q mismatch in 15 patients with cirrhosis and various degree of hepatocellular dysfunction, and all of them are smokers 12. They assessed the hypoxic response after patients breathe oxygen with various concentrations. They found that mild pulmonary vasodilatation and hypocapnia are accompanied with a slight right shift of the oxyhaemoglobin dissociation curve under the baseline, however the levels of TLCO and PaO 2 are normal in these patients. In addition, the distributions of VA/Q ratios display mild abnormalities of VA/Q relationships: a small proportion of the cardiac output perfused regions with a low VA/Q ratio. Shunt is conspicuously absent. After patients breathe 11% oxygen, pulmonary artery pressure and vascular resistance are increased in the club, while the VA/Q distributions remain stable. The capacity of oxygen diffusion has no change, and PaO 2 levels have no signifcant difference from the predicted ones. On the contrary, when these patients breathe 100% oxygen, intrapulmonary shunting is prominently detected.In addition, VA/Q mismatch is worsened without the dramatical haemodynamic changes.Interestingly, patients with two or more cutaneous spider naevi, regardless of the presence of other associated stigmata, show the greater liver dysfunction, the lower systemic and pulmonary vascular resistance, the less hypoxic vascular response, the lower PaO 2 levels,and the more VA/Q mismatch than the individuals without spider naevi. In fact, anastomoses between arteries and veins on the pleural are more common than dilated intrapulmonary capillary in patients with chronic liver disease. Spider naevi maybe a potential useful clinical marker to predict vascular disorder in HPS.
VA/Q mismatch can be improved by hypoxic vascular response, which is also present in patients with primary pulmonary hypertension, including idiopathic pulmonary fibrosis 23, 24.HPV induces pulmonary vascular constriction in response to alveolar hypoxia, predominantly in arteriole (less than 500 μm in diameter) 25.
Melot and their colleagues explored the role of intrapulmonary and extrapulmonary factors in PaO 2. Hypoxemia is associated with the mild to moderate degrees of VA/Q mismatch and intrapulmonary shunting, both of which contribute equally to hypoxemia 26, 14. Several evidence shave revealed that patients with cirrhosis present the mild limitation of oxygen diffusion,indicating a potential role of impaired diffusion in the pathogenesis of hypoxemia. By using the technique of multiple inert gas elimination, Castaing and Manier found that intrapulmonary shunting, VA/Q mismatch and limitation of oxygen diffusion are concurrent in patients with cirrhosis 27. By using the same technique, Agusti and associates confirmed that exercise would not improve gas exchange abnormalities in patients with cirrhosis 28. In addition, extrapulmonary factors, including minute ventilation and cardiac output, are also important in modulating oxygenation in lung. High minute ventilation and high cardiac output can reverse the effect of VA/Q mismatch at rest which induce hypoxemia and remains the alveolar PaO 2 and mixed venous PaO 2 in the normal levels 29,30. High cardiac output, a common haemodynamic feature in patients with HPS 31, has been regarded as one important mechanisms controlling the compensation of gas exchange abnormalities in HPS 32.
Angiogenesis in HPS
Recently, more and more studies suggest that the pathophysiology of HPS may involve some other factors in addition to IPVD. Early studies found the increased pulmonary capillary density in the microvasculature during cirrhosis 4, while more recent studies identified angiogenesis in the splanchnic and hepatic vasculature mediated in part by vascular endothelial growth factor(VEGF-A) which has been recognized as a key component in the development of a collateral circulation 35. Together, these observations raise the possibility that angiogenesis may contribute to the development of HPS.
Diseased liver produces and releases cytokines and growth factors that diffuse into the lung and then are targeted to specifc vascular receptors located on the endothelial cells within nearby blood vessels. Activated endothelial cells enter the cell cycle to do both cell proliferation and migration. Furthermore, sprouting endothelial cells roll up to form a tubular vessel. Meanwhile,adhesion molecules contribute to the forward vessel sprouting and remoulding around the vessel.Finally, vascular SMCs and pericytes provide a structural support, thus stabilizing the newly formed vessel tubes.The switch to angiogenic phenotype is dependent on the change of local concentration of pro-angiogenic factors and anti-angiogenic factors. Pulmonary angiogenesis, the growth process of new vessels and capillary networks, has been observed by CT angiogram in CBDL rats, as shown in Figure 3.

Figure 3. Representative CT angiogram (left panel). Note that there are fewer resistance Pas (0.1-0.2 mm,yellow), but more pulmonary alveolar capillaries (red) in the lungs of CBDL rats than those of sham rats.Right panel: quantifcationof vessel count, binned by diameter (bin width: 0.1 mm). From Thenappan, T, Am J RespirCrit Care Med, 1535-4970
Furthermore, the increased capillary networks facilitating gas exchange abnormalities are precisely defined in the experimental HPS. It has been confirmed that microvessel counts are directly correlated with gas exchange abnormalities after CBDL, and the inhibition of angiogenesis can improve gas exchange and intrapulmonary shunting, supporting the hypothesis that angiogenesis contributes to HPS. Mechanisms underlying this effect have been investigated in HPS. Anatomic right to left shunting through angiogenic pulmonary vessels or alterations in caliber or permeability in the pulmonary neovasculature can impair the diffusion of oxygen from the alveolus into alveolar capillaries. Moreover, there are bronchial nutrient circulation and pulmonary functional circulation in the pulmonary vasculature. The angiogenic response may include one or both of these vascular beds. In the bronchial circulation, angiogenesis is responsible for a basal component of anatomic right to left shunting, which is also anticipated to increase anatomic shunting.
Hypoxia pulmonary vascular remodeling (HPVR)
Circulating factors released from dysfunctional liver and hypoxic conditions (induced by IPVD and intrapulmonary shunting) influence the pulmonary vasculature by facilitating the hypertrophy of the vascular media and the invasion of non-musculararterioles by SMCs,which is involved in pulmonary vascular remodeling. This indicates that it could be a key pathophysiological component in HPS.
High-altitude population exhibits the increased pulmonary artery pressure due to vascular remodeling because the administration of oxygen fails to reverse hypoxemia 38. It is supported by the autopsy studies demonstrating medial hypertrophy and muscle extension into distal arterioles.
In order to further prove the concept that an exposure to chronic hypoxia can lead to apparent alteration in pulmonary arteries, researchers conducted a simulated climb of Mount Everest 39. In this study, volunteers staying at this environment present the reduced levels of hypobaric hypoxia over a period of 6 weeks. Catheterization has been placed when volunteers are at rest and on exercise after exposure to progressively higher altitudes. Compared with the volunteers with the condition of acute hypoxia before the climb, pulmonary arteries pressures of the volunteers underlying chronic hypoxia are significantly increased after 40 days. Interestingly, there is a limitation of vasodilator response to the acute administration to 100% oxygen. Therefore, all these fndings suggest that signifcant structural remodeling in the pulmonary vascular bed is induced in response to chronic hypoxia 40. Thus, chronic hypoxia induced by gas exchange abnormality may be a key factor to contribute the subsequent development of pulmonary hypertension in HPS.
Histological evidence of vascular remodeling is also available from the lung tissue derived from the hypoxia animal model. The lungs of these animals exhibit the arteriolar muscularization,the increased intimal thickness and the reduced lumen area. The degree of the alteration in pulmonary vessel wall is dependent on the species type and the animal sex 41, 42. Structural changes in vessel wall include the appearance of SM-like cells, the elevated expression of SM-actin antigen in non-muscularized vessels close to alveolar wall, which is indispensible for pulmonary hypertension after exposure to hypoxia. The thickening in medial vessel is linked to the hypertrophy and the abnormal proliferation of SMCs, as well as the increased accumulation of extracellular matrix proteins. In addition, the thickening in adventitial vessel is mainly attributed to the transition of cell type from fibroblasts to myofibroblasts. Subsequently, activation of myofbroblasts results in the deposition of extracellular matrix protein 43, 44. The alteration of vessel intima has often been observed in the pathogenesis of hypoxia-induced pulmonary hypertension in animal model 45. In 2011, Thenappan and his coworkers suggested that a pulmonary vascular pathology consists of the medial thickness in vessel and the loss of lumen (Figure 4A). This identification may be suitable in some cases, but not in all cases. Interestingly, the increased medial thickness of resistance pulmonary arteries is also observed in the lung of CBDL rats (Figure 4B and 4C) 46.

Figure 4. Proliferative, obstructive vasculopathy of the resistance pulmonary arteries observed in CBDL rats.(A) Representative micrographs of hematoxylin and eosin (H&E) staining of the resistance pulmonary arteries in CBDL and sham animals. (B, C) Representative images and mean data showing the increased % medial thickness of small pulmonary arteries in HPS, which is measured by using immunofluorescent images co-stained with smooth muscle actin (red) and von Willebrand factor (vWF, green). From Thenappan, T, Am J RespirCrit Care Med, 1535-4970
In recent years, some studies in this fled have also alleged that the cross-sectional area of the pulmonary vascular bed is decreased, due to the loss of small blood vessels following the treatment of chronic hypoxic 47-49. As described above, pulmonary vascular remodeling is an important structural or non-vasoconstrictive component of chronic hypoxia-induced pulmonary hypertension. But it has been demonstrated that angiogenesis in pulmonary capillaries emerges with the administration of hypoxia, which strongly challenges the phenomenon of vascular rarefaction 50, 51. Considering that angiogenesis can reduce the perfusion resistance in the pulmonary vascular bed, angiogenesis is assumed to antagonize pulmonary hypertension. This hypothesis has been supported by the fact that the upregulation of angiostatin in lung aggravates the symptom of pulmonary hypertension in the chronically hypoxic mice. Meanwhile, the upregulation of VEGF in lung has been reported to ameliorate hypoxic pulmonary hypertension 52.However, angiogenesis, as well as the upregulation of VEGF in CBDL rat serum, have also been observed in the CBDL rat. Thus, the effects of angiogenesis and VEGF in the subsequently hypoxia-induced pulmonary hypertension need further investigation.
Portopulmonary hypertension
The syndrome of portopulmonary hypertension (POPH) is similar to the hypoxia-induced pulmonary hypertension in HPS described above, both of which increase the pulmonary arterial pressure. However, the pathogenesis of POPH is still unknown. Actually, POPH and HPS are two frequent complications of liver diseases, with prevalence around of 6% and 10% respectively among liver transplantation (LT) candidates. Both conditions result from a lack of hepatic clearance of vasoactive substances produced by visceral organs. Subsequently, these substances mainly cause PVR and some degrees of vasoconstriction in POPH, such as the elevated pulmonary arterial pressure, the right ventricular dysfunction and the right heart failure.
Unfortunately, because of the short research period, the suitable POPH animal model is unavailable. Thus, pathogenesis of POPH is still unclear. Furthermore, most knowledge about the pathogenesis of PPHT is mainly based on the research related to pulmonary artery hypertension(PAH). Interestingly, POPH and PAH have some similar in histological features identified by the stenosis of the pulmonary arterial lumen. In general, the pathological changes result in the thickening in pulmonary arterial walls and the narrow blood vessel, both of which lead to the aggravation of PVR 53, 54.
A concept that POPH is caused by an imbalance between vasoconstrictors and vasodilators promoting pulmonary vasoconstriction has been proposed. The hyperdynamic circulation stimulates the development and aggravation of POPH. Due to the increased pulmonary blood flow, the elevated shear stress may induce the injury and dysfunction in endothelial cells accompanying with the progressive PVR. However, it still needs further studies to support the idea that some substances, generated by the dysfunctional liver or metabolized by the healthy liver, can enter the pulmonary circulation through portosystemic shunts and thus affect the pulmonary circulation. Some researchers hypothesize that these substances include endothelin-1A (ET-1A), tromboxane A2, interleukin-1 (IL-1), interleukin-6 (IL-6), angiotensin-1, glucagon and serotonin, among which the ET system is the best studied 55-58. Previously, it has been demonstrated that the ET-1 levels are upregulated in patients with POPH, compared to patients with cirrhosis but not POPH 54.
The question about whether the transition and coexistence between HPS and POPH are present is still unclear. Some studies have proposed the possibility of transition between HPS and POPH 59-61, or coexistence of HPS and POPH 62. To explain this issue, two reasons have been provided: one is the dysregulation of a common vascular signaling pathway, which may lead to pulmonary vessel vasoconstriction or vasodilation; the other is preferential binding of ET-1 to either the ET-1A or the ET-1B receptor, thus leading to vasoconstriction or vasodilation respectively 59.
Hemodynamic alterations in HPS
In general, liver cirrhosis and portal hypertension have been reported to promote a hyperdynamic circulatory syndrome, accompanied with the high cardiac output, the increased heart rate, the reduced total systemic vascular resistance (SVR) and the decreased arterial pressure 63. This syndrome contributes to the generation of sodium retention, ascites and renal impairment of cirrhosis 64, all of which have great prognostic value 65. Moreover, the dysfunction of circulation is directly affected by the severity of cirrhosis, which can be predicted by the Children score and the presence of ascites 66. Unfortunately, the hyperdynamic circulatory syndrome does not appear at any other conditions, except in the supine position 67. The high cardiac output and the decreased SVR are consistent with primary volume expansion, as suggested by the various theories of ascites formation and vasodilatation in vessel wall thus resulting in the activation of sodium retention and compensatory increase of blood volume 64. And the latter issue is only suitable to explain the decrease in arterial pressure which usually occurs in patients with cirrhosis.
TabIe 3. Haemodynamic profIes of patients with positive and negative contrast echocardiograms (CE)FromDe, B. K, J Gastroenterol Hepatol, 0815-9319

Normally, HPS occurs in patients with cirrhosis, but not all cases. Binay and his colleague did the haemodynamic studies in HPS patients 68. Three patients with positivecontrast echocardiograms (CE) and 24 patients with negative CE have been studied. After an overnight,haemodynamic investigations were carried out by using standard procedure. They found that the mean pulmonary arterial pressure and pulmonary capillary wedge pressure in the positive group are lower than those in patients with negative CE, and the other haemodynamic indicators have no signifcant differences, as shown in table 2. The fnding indicates that the status of heamodynamic is really changed in HPS patients.
In addition, advanced liver dysfunction associated with hyperdynamic circulation syndrome has been proposed to be a key factor for the HPS generation 12. But it has also been reported that congenital hepatic fbrosis and portal vein thrombosis occur in HPS. Thus, it is valuable to investigate whether portal hypertension can contribute to the development of HPS. Moreover, if hepatic dysfunction is the only requirement for the HPS development, HPS should predominantly occur among child's class C patients which represent the highest levels of hepatic dysfunction.However, Abrams and his coworkers reported that 15 of 25 cases with HPS patients are child's class A, while two of them are child's class C. In the study conducted by Binay mentioned above, 2 of the 3 HPS cases are Child' class B, while one is Child's class C 69, and the one case of subclinical HPS is Child's class A. In these studies reporting the low values, most of them only investigated the patients with advanced cirrhosis so that the data investigating haemodynamic assessment in HPS patients is missing. Importantly, the alteration of haemodynamic profles in HPS may also be effected by other factors, such as angiogenesis, hypoxia-induced pulmonary vascular remodeling and POPH.